Chip发表南京电子器件研究所光量子器件团队最新成果:基于半导体光量子芯片的经典-量子共纤传输多用户纠缠分发网络
FUTURE远见| 2024-06-14
Future|远见
Future|远见future选编
近日,南京电子器件研究所牛斌研究员联合南京师范大学、安徽大学、南京大学等团队以「Coexistence of multiuser entanglement distribution and classical light in optical fiber network with a semiconductor chip」¹为题在Chip上发表研究论文,利用半导体布拉格反射波导直接产生的偏振纠缠构建了与经典光同传的三用户量子密钥分发网络,并实现了基于香农置乱扩散架构的图像加密和量子秘密共享等应用。第一作者为经旭、钱铖、郑晓冬,通讯作者为陆亮亮、牛斌、江东、盛冲和刘奕辰。Chip是全球唯一聚焦芯片类研究的综合性国际期刊,是入选了国家高起点新刊计划的「三类高质量论文」期刊之一。
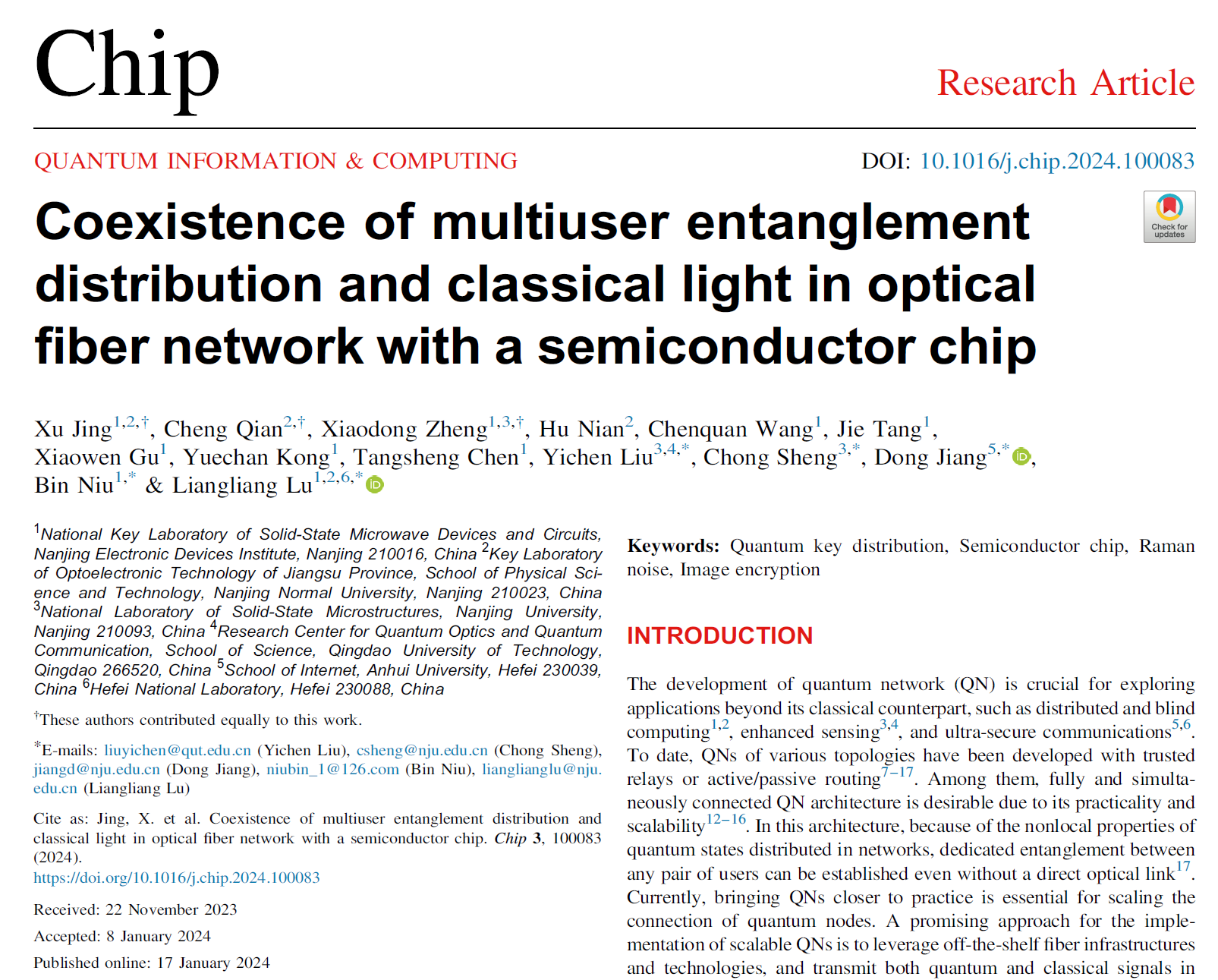
量子通信发展的主要目标是实现大规模的网络部署,借助现有经典光纤网络设备,将量子信号与经典信号共纤传输,结合可扩展的网络架构,是实现该目标的重要途径之一。经典-量子信号共纤传输面临的主要挑战在于高功率经典光引发的强噪声问题,这会导致信道容量降低,进而成为量子通信速率和距离的限制因素。尽管在光纤量子密钥分发网络中已经取得了一系列经典-量子信号共存的成果,但这些研究主要集中在点对点的系统模型,多用户全连接纠缠分发网络与经典光的共存应用尚未得到充分挖掘。因此,设计具有高噪声抵御能力的共纤同传方案对于推动大规模量子纠缠分发的网络化应用至关重要²。
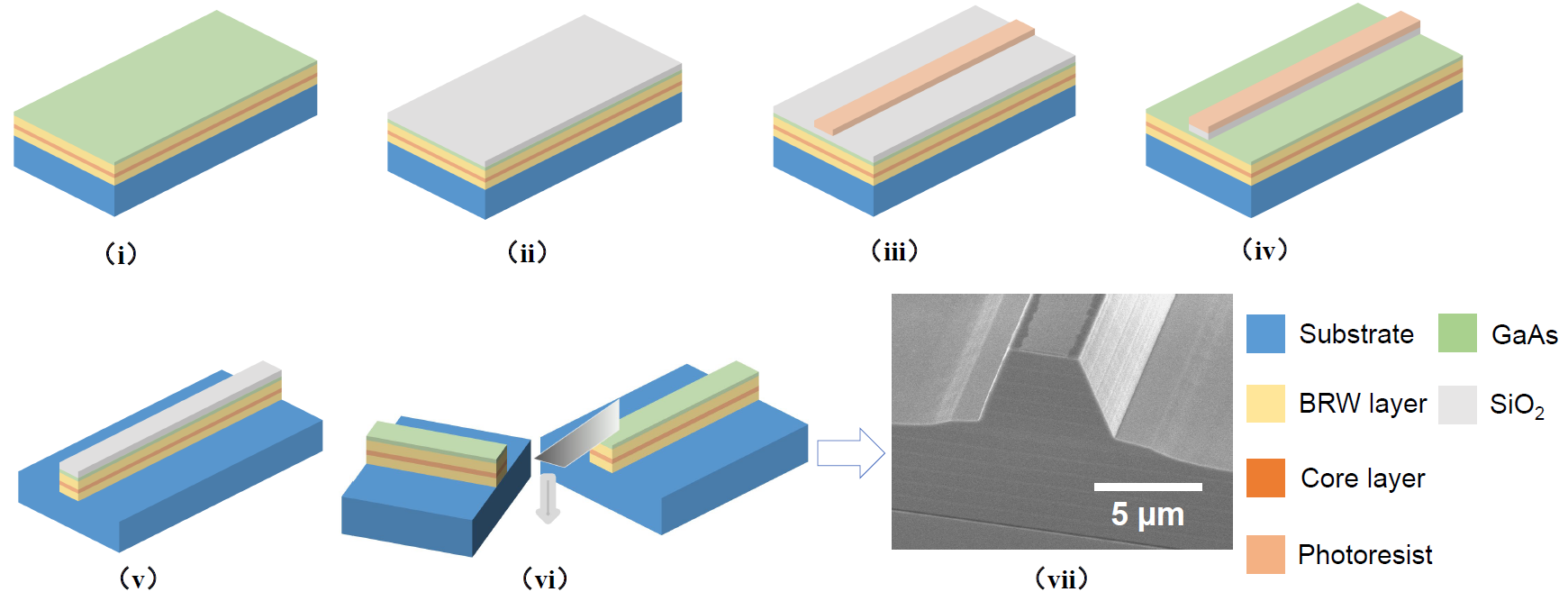
图1 布拉格反射波导量子光源制备流程图。(i) 样品生长。(ii) 刻蚀掩膜生长。(iii) 光刻胶掩膜光刻。(iv) 氟基刻蚀SiO₂掩膜。(v) 氯基刻蚀AlGaAs掩膜。(vi) 波导端面解理。(vii) 样品实物图。
研究团队设计并制备了一个具有高模式重叠度的AlGaAs半导体光量子芯片(图1),通过布拉格反射波导结构中的模式相位匹配直接产生了宽带偏振纠缠³。该量子光源在42 nm带宽下的偏振纠缠态保真度保持在96%以上,亮度达到了1.2×10⁷ Hz/mW。
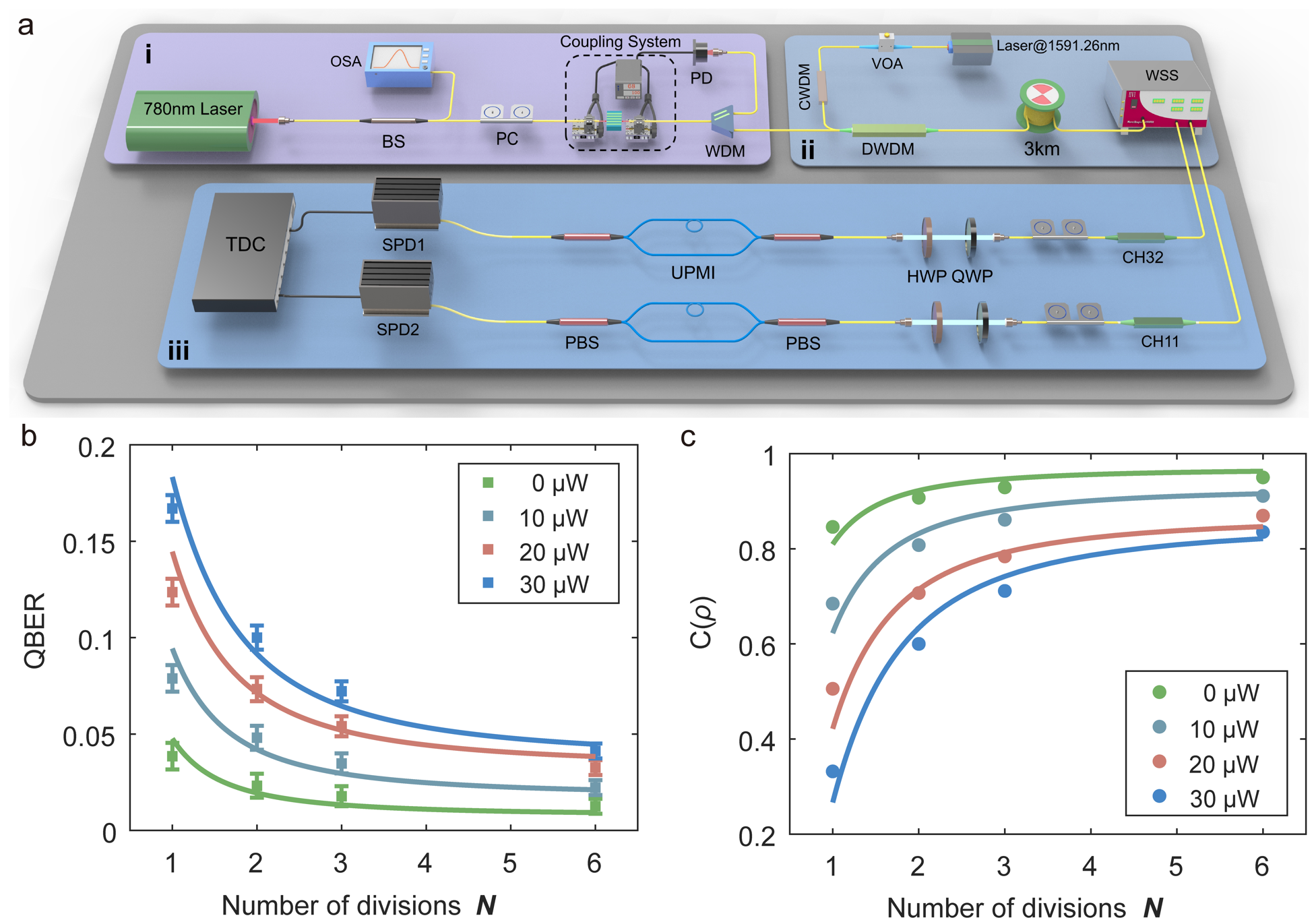
图2 实验装置图和结果图。a. 经典-量子共纤传输噪声影响分析实验示意图。b、c分别描述了在给定经典光功率下,Z基和X基的平均量子比特误码率(b)和 I-concurrence (c)随不同频率维数的变化。
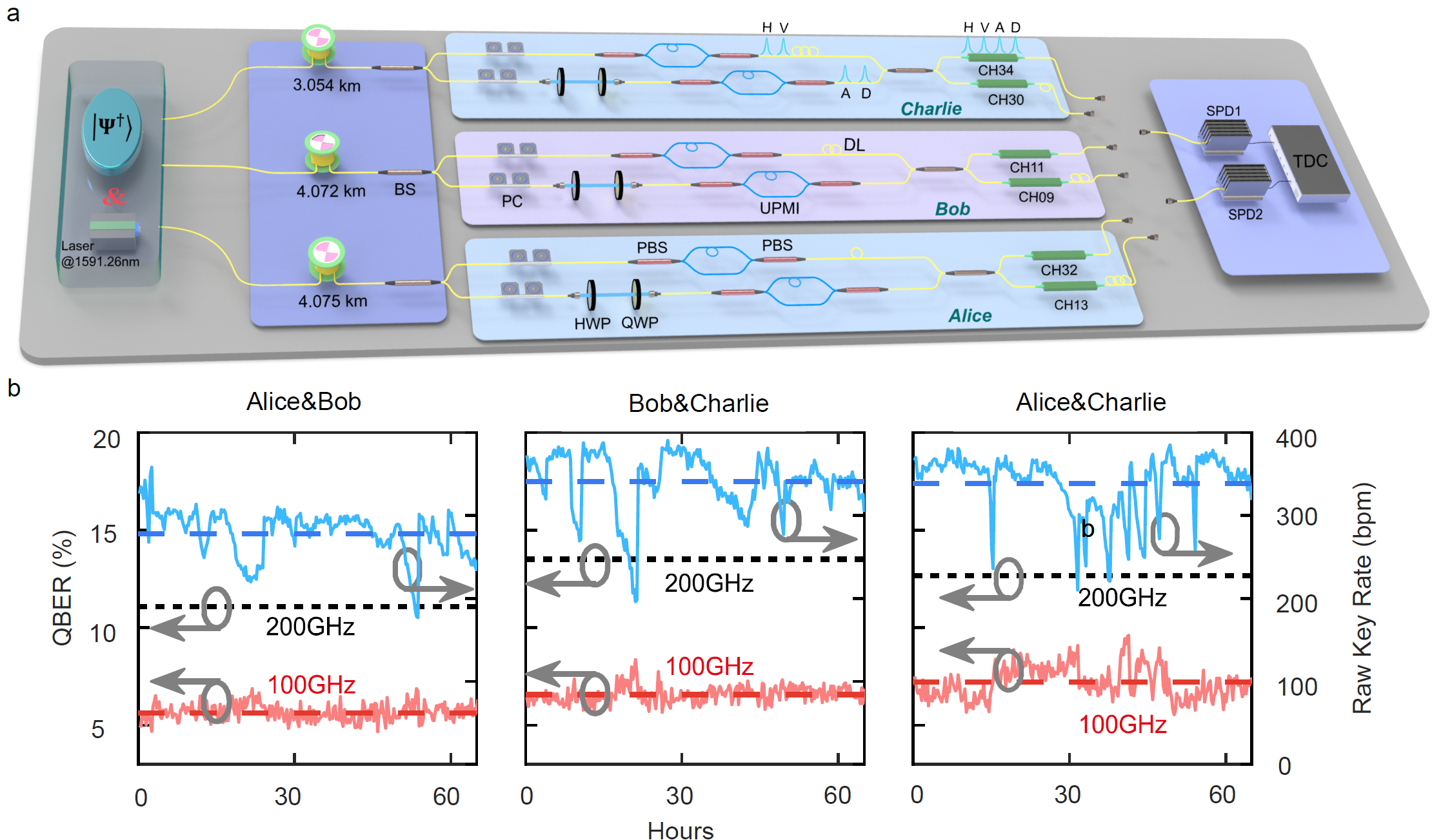
图3 经典-量子共存的三用户量子纠缠分发示意图。
基于产生的偏振-频率超纠缠,团队验证了利用超纠缠的高维子空间编码能够有效地抵御共纤系统的拉曼噪声(图2)。随着频率维数N的增加,平均量子比特误码率(纠缠度)会显著降低(增加),这意味着利用频率关联可以有效抑制经典噪声的影响。随后,研究人员利用波分复用技术选择出三对光子并与经典光组合分发给三个用户,成功构建了三用户量子通信网络(图3),可在任意用户之间通过BBM92通信协议实现量子密钥分发。实验中,结合两个不等臂的偏振保持干涉仪和时分复用技术,每个用户只需要一个探测器即可实现成码,这将极大地降低系统的成本,具有重要的实际应用价值。最后,该团队通过使用生成的无条件安全密钥,实现了对每对用户之间的图像进行加密和解密以及三用户量子秘密共享。其中图像加密基于香农置乱-扩散体系结构(SCDA),使用生成的量子密钥对原始图片完成多轮置乱和扩散操作实现图片加密。和传统直接XOR运算加密方式不同,该方案可抵御专门针对图像、视频等多媒体数据的裁剪攻击策略(图4)。
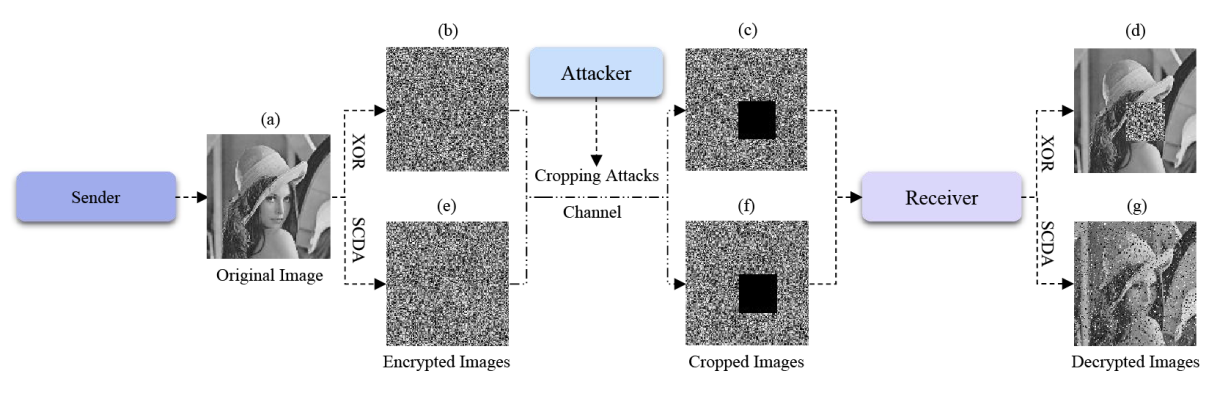
图4 抗裁剪攻击的比较。
该芯片化的偏振纠缠光源具有尺寸小、光子频谱范围宽和可扩展空间大等优点。未来可将具有高非线性、直接带隙结构的AlGaAs布拉格反射波导与分布式反馈激光器结合实现单片集成的常温电泵浦宽带偏振纠缠光源,利用便携式、易操作、低成本的解码模块,从而构建大规模的量子通信网络。该工作为极强经典信号与纠缠信号的同纤传输提供了新的思路和方法。
Coexistence of multiuser entanglement distribution and classical light in optical fiber network with a semiconductor chip¹
The main goal of quantum communication development is to achieve large-scale network deployment, and co-fiber transmission of quantum signals with classical signals using existing classical fiber network equipment combined with scalable network architecture is one of the important ways to achieve this goal. The main challenge of classical-quantum signal co-fiber transmission is the strong noise caused by high-power classical light, which leads to the reduction of channel capacity and then becomes the limiting factor of quantum communication rate and distance. Although the coexistence of classical-quantum signals in fiber quantum key distribution networks have been deeply investigated, these studies mainly focus on point-to-point link, and the applications of multi-user fully-connected entangled distribution networks coexisting with classical light have not been fully explored. Therefore, the design of co-fiber transmission schemes with high noise resilience is crucial for promoting networked applications of large-scale quantum entanglement distribution².
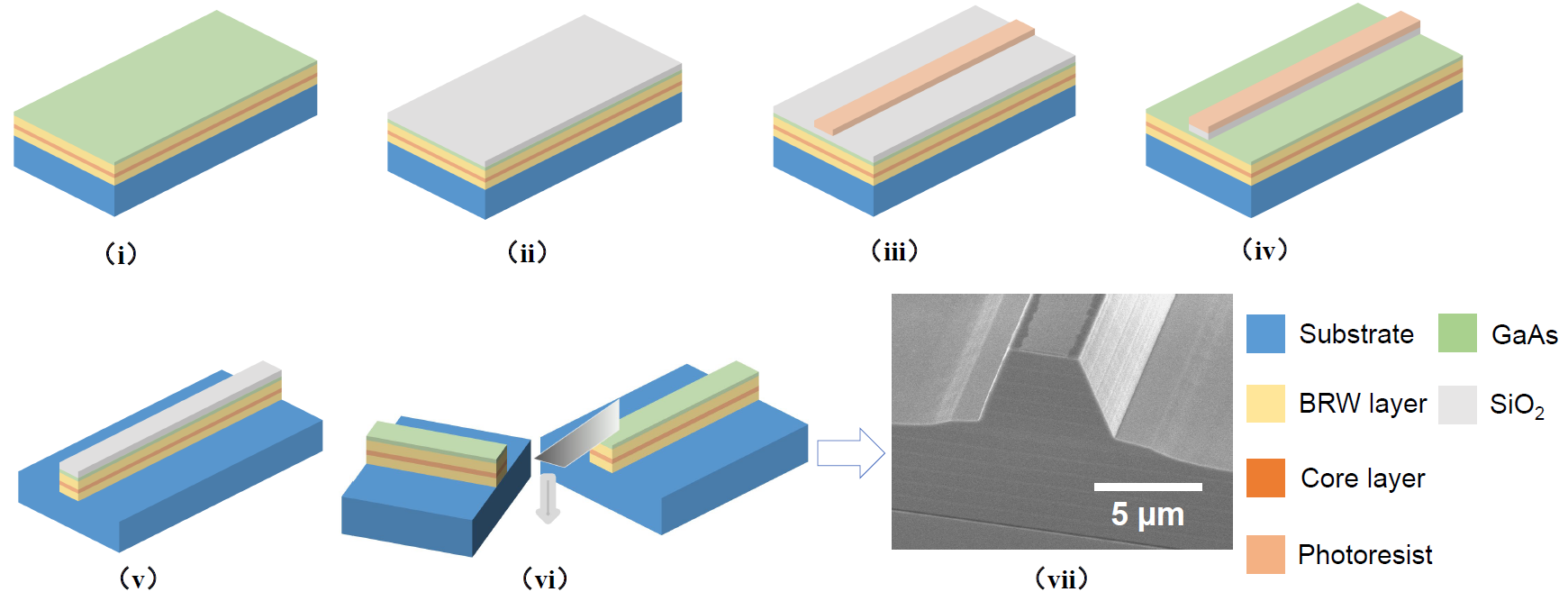
Fig.1 | Six-step fabrication process of the BRW source. (i) The device is fabricated on a semi-insulating GaAs substrate. BRW layers are grown on the chip by molecular beam epitaxy. (ii) A thickness of 1 μm SiO₂ cladding is deposited via plasma enhanced chemical vapor deposition. (iii) The waveguide pattern is defined by lithography along GaAs crystal orientation [110]. (iv) SiO₂ is etched away in a reactive ion etching step with fluorine gas with patterned photoresist as etching mask. (v) Then AlGaAs waveguide ridge was etched by carefully tuning chlorine gas to ensure the planeness of the sidewall. (vi) Finally, the sample is cut along the cleavage plane with smooth edges. (vii) The scanning electron microscope image of the fabricated BRW sample.
The research team has manufactured an AlGaAs semiconductor chip with high mode overlap (Fig.1), which directly generates broadband polarization entanglement through mode phase matching in the Bragg reflection waveguide structure³. The polarization entanglement fidelity of the single chip source remains above 96% at a bandwidth of 42 nm, and the brightness reaches 1.2 × 10⁷ Hz/mW.
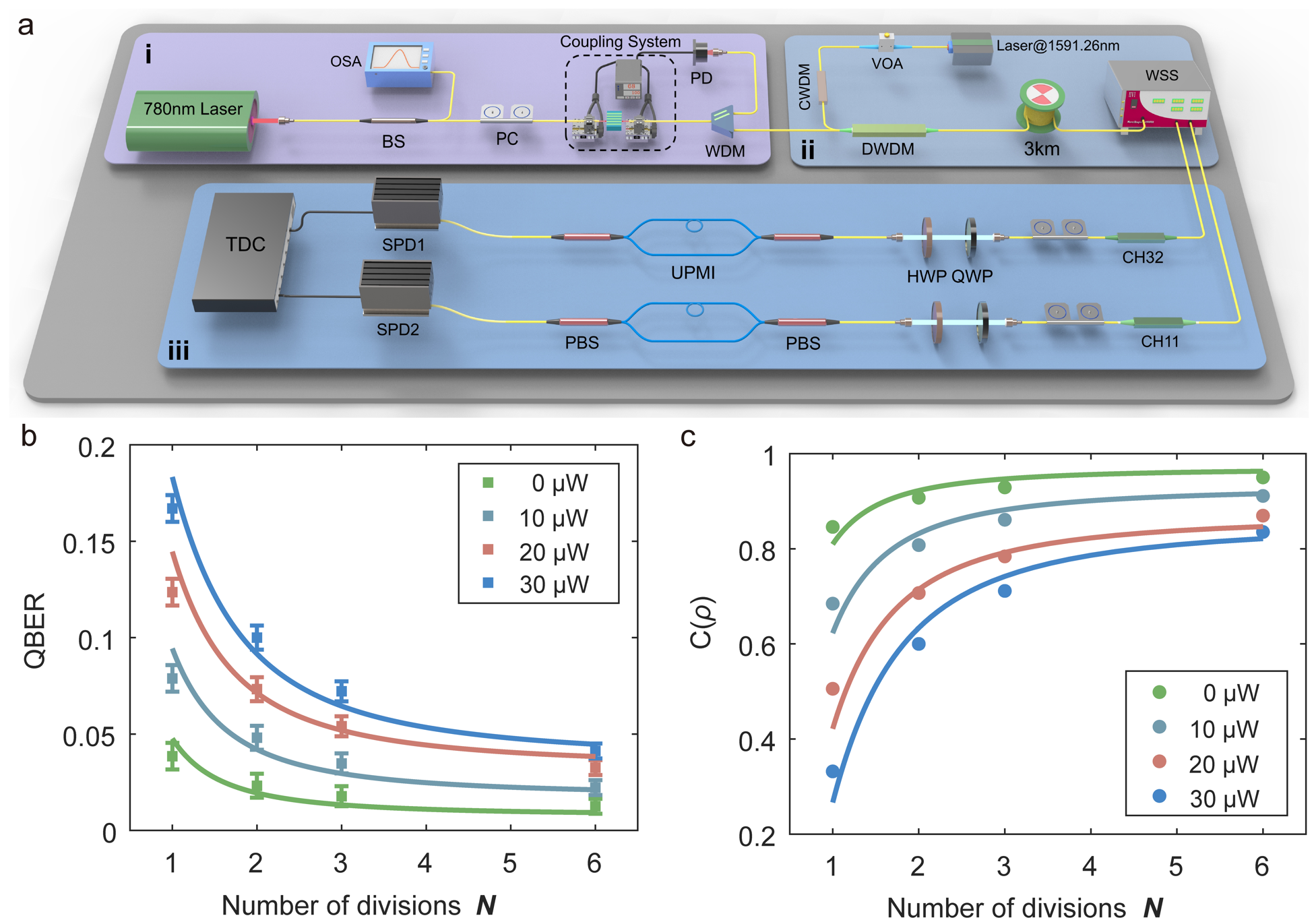
Fig.2 | Experimental setup and main results of noise resilience. a, the schematic of experimental setup, containing three parts: photon-pair source, classical–quantum link and wavelength selective switch (WSS), and polarization analysis module. b, c, respectively depict the averaged error rates and I-concurrence with different frequency-bin number against the classical signal power. The solid lines represent the theoretical curves.
The generated polarization-frequency hyperentanglement is employed for the characterization of its increased resilience to noise. In experiments, the noise is introduced by coexisting a classical light with quantum signals in the same fiber, which is extremely practical for installed fiber networks (Fig.2a). It has been demonstrated that the Raman noise leads to accidental coincidences in experiments and can be equivalently divided by the frequency subspace dimension N. By choosing appropriate frequency channels, the probability of measuring false coincidences between noise-noise photons or noise-signal photons is reduced, while the probability of measuring a true coincidence between correlated photons is essentially unchanged. Fig. 2b shows the averaged quantum bit error rates (QBERs) in Z and X bases of various division number for different classical power. Fig. 2c gives the lower bounded I-concurrence calculated as a function of division number with four classical light powers. The results show that as the number of mutually exclusive subspaces increases, QBERs decrease dramatically, especially over highly noisy quantum channels, indicating stronger noise resilience for larger N in quantum networks. Therefore, the additional quantum correlation in frequency can effectively overcome physical noise and increase the channel capacity, even under the extreme noise conditions that would otherwise preclude quantum communication. Furthermore, a fully connected three-user noisy network (Fig.3) has been demonstrated by multiplexing 3 pairings of DWDM channels so that polarization entanglement can be shared between each possible two-party link. By applying time-multiplexing in the polarization analysis module, the number of single photon detectors required has been reduced to one per user on the network. The team subsequently utilized the generated unconditionally secure keys to successfully demonstrate point-to-point image encryption and decryption, as well as three-user quantum secret sharing on the deployed quantum network. The image encryption is based on the Shannon Confusion-Diffusion Architecture (SCDA), utilizing the generated quantum keys to perform multiple rounds of confusion and diffusion operations on the original image to achieve encryption. In contrast to the conventional direct XOR encryption method, this approach provides resilience against cropping attacks and other attack strategies specifically targeting images, videos, and other multimedia data (Fig.4).
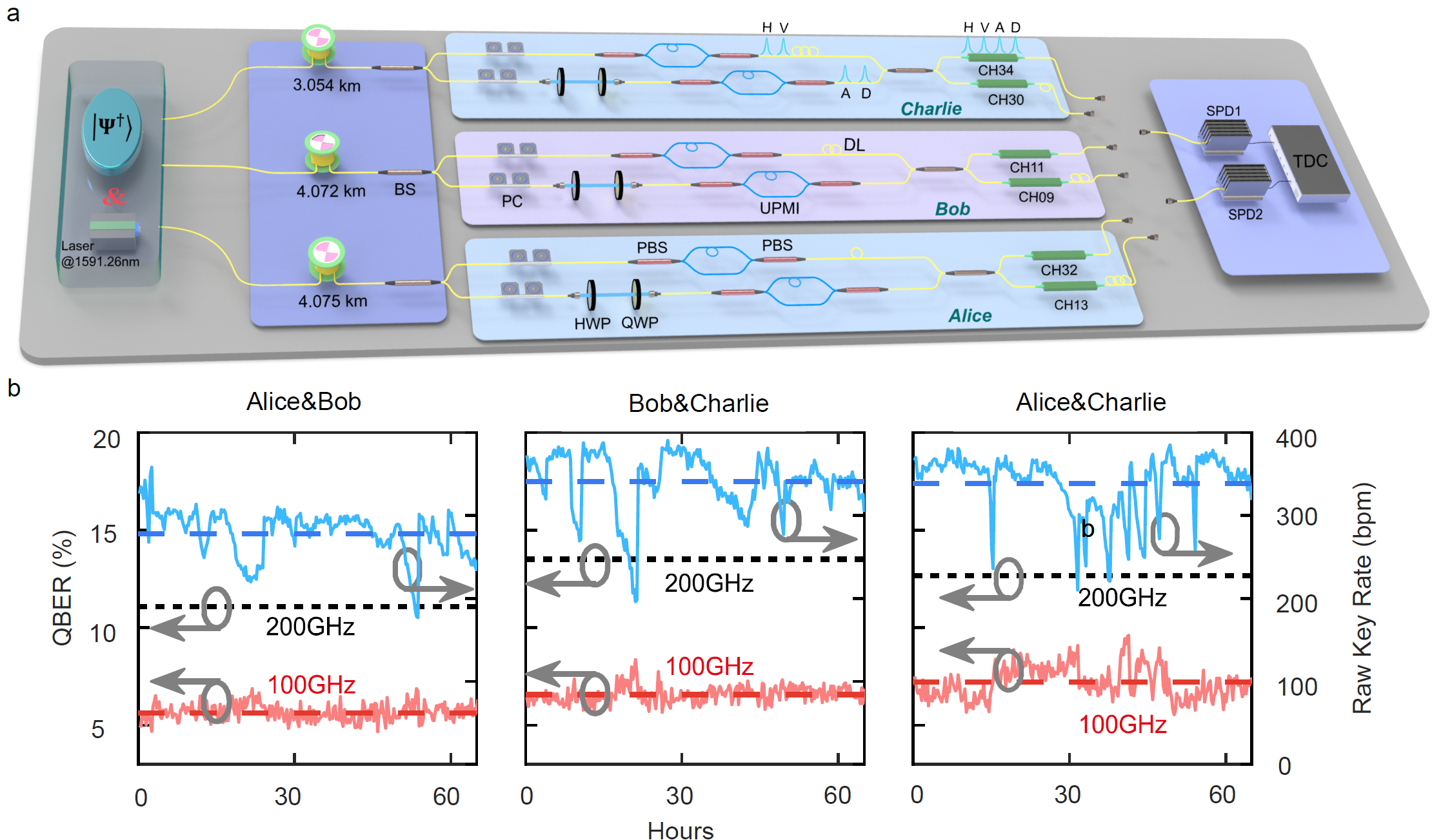
Fig.3 | Scheme of multiuser quantum entanglement distribution over noisy fiber networks.
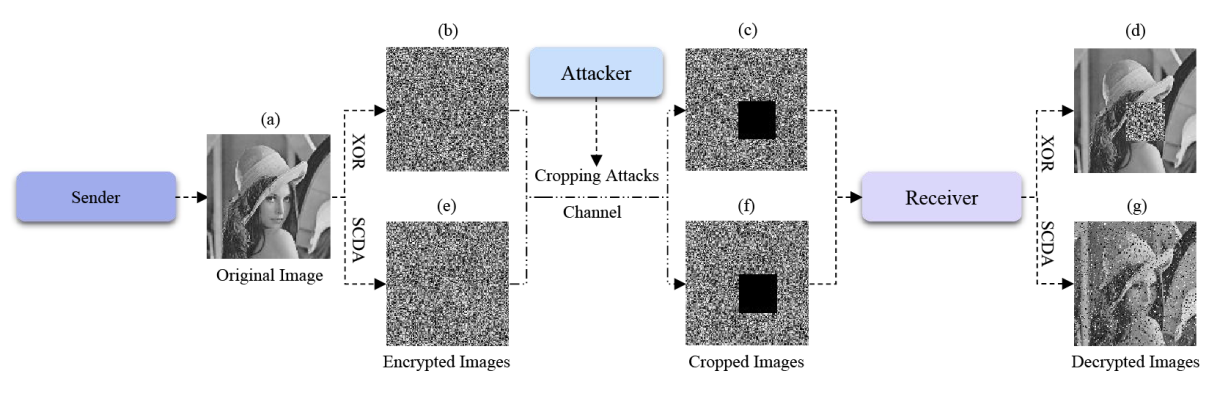
Fig. 4 | Comparison of resistance against cropping attacks.
The combination of broad spectrum and considerable brightness makes this entanglement source highly promising for large-scale, wavelength multiplexed, and fully connected quantum networks. This work paves the way for multi-user quantum communication with integrated photon architectures compatible with real-world fiber optic communication networks.
参考文献
1. Jing, X. et al. Coexistence of multiuser entanglement distribution and classical light in optical fiber network with a semiconductor chip. Chip 3, 100083 (2024)
2. Thomas, J. M. et al. Designing noise-robust quantum networks coexisting in the classical fiber infrastructure. Opt. Express 31(26) 43035-43047(2023).
3. Helmy, A. S. et al. Phase matching in monolithic Bragg reflection waveguides.Opt. Lett. 32, 2399 (2007).
论文链接:
https://www.sciencedirect.com/science/article/pii/S2709472324000017
作者简介
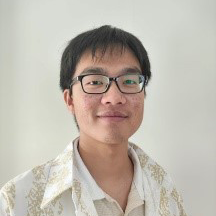
经旭,南京师范大学物理科学与技术学院在读硕士研究生,主要研究方向为
集成光量子芯片的制备与应用。
Xu Jing is a master student at the School of Physics and Technology of Nanjing Normal University. Currently, he is working on the fabrication and application of integrated photonic quantum chip.
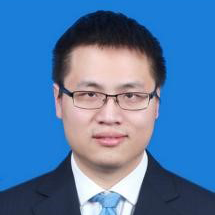
牛斌,南京电子器件研究所研究员级高级工程师,2008年本科毕业于华中科技大学,2013年博士毕业于中国科学院半导体研究所。主要研究方向涵盖GaAs、InP基化合物部半导体光子器件与太赫兹器件,近期他的研究专注于AlGaAs量子光源及光量子集成平台。
Bin Niu is the researcher level senior engineer of Nanjing Institute of Electronic Devices. He has recieved his bachelor’s degree from Huazhong University of Science and Technology in 2008, and Ph.D. from Institute of Semiconductor of the Chinese Academy of Sciences in 2013. His research interests include GaAs, InP based semiconductor photonic and terahertz devices. Recently, his research focussed on AlGaAs quantum source and quantum photonic integration platforms.
关于Chip
Chip(ISSN:2772-2724,CN:31-2189/O4)是全球唯一聚焦芯片类研究的综合性国际期刊,已入选由中国科协、教育部、科技部、中科院等单位联合实施的「中国科技期刊卓越行动计划高起点新刊项目」,为科技部鼓励发表「三类高质量论文」期刊之一。
Chip期刊由上海交通大学出版,联合Elsevier集团全球发行,并与多家国内外知名学术组织展开合作,为学术会议提供高质量交流平台。
Chip秉承创刊理念: All About Chip,聚焦芯片,兼容并包,旨在发表与芯片相关的各科研领域尖端突破性成果,助力未来芯片科技发展。迄今为止,Chip已在其编委会汇集了来自14个国家的69名世界知名专家学者,其中包括多名中外院士及IEEE、ACM、Optica等知名国际学会终身会士(Fellow)。
Chip第三卷第二期已于2024年6月在爱思维尔Chip官网以金色开放获取形式(Gold Open Access)发布,欢迎访问阅读本期最新文章。
爱思唯尔Chip官网:
https://www.sciencedirect.com/journal/chip